Frederick Lanchester
We all understand that the primary purpose of the suspension system is to minimise the transmission of road shocks to the car's occupants - but the very earliest automobiles were un-sprung, or had a rudimentary suspension system at best. Pioneer designer Frederick Lanchester took elaborate pains to perfect a spring system that ensured his car moved gently up and down on its suspension at the same rate as a persons body moves up and down when they walks - a natural function that was emulated in the hope of avoiding any disturbance of the body's mechanism.
But calculating spring rates to create the desired effect was difficult, as you needed to factor
tyres and even the seat cushion - and as late as the 1960's some cars had seat springs that would resonate in sympathy with the road springs - not ideal. Designers soon discovered, however, that suspension was not just a means of creating a more comfortable ride for the occupants of the
automobile - as it played an almost as important part in protecting the car itself from the variations in road surface, and an even more important role in maintaining contact between the
tyres and the road so that control could be exercised in braking, accelerating and most importantly, when cornering.
Creating the first suspension systems that actually worked was a matter of proportion, not of quantity: of creating the correct ratio of sprung to unsprung weight - and that is why the nature of the linkages or other mechanisms whereby the wheels are located and supported has to be considered along with the characteristics of the springs themselves. In the earliest cars, the suspension systems used were generally derived from those of horse-drawn vehicles. Even in those days it was usual for the springing to be interposed between the axles and the chassis of the car.
Some horse-drawn carriages had the chassis frame unsprung - mounted directly on the axles - and only the body was carried (or suspended) on springs. However, the pioneer designers appreciated that such a layout was not practicable for the motor car: control was to be effected not through flexible reins but through various mechanisms for which the relative movement of a sprung body would have been at least inconvenient. Leaf springs of tempered steel strip soon became the most common suspension media, but quite soon examples appeared of helical (commonly miscalled 'coil') springs. The final form of steel spring was the torsion bar, its application to the motor car being patented by Ferdinand Porsche and widely used after
1950 when the patents expired.
Ferdinand Porsche's Torsion Bar Suspension
The torsion bar is a steel rod which is clamped to the chassis at one end and has a lever at the other end connected to the wheel; movement of the wheel causes the bar to be twisted, and its resistance to this twisting (or torsion) provides the springing. The helical spring is in fact merely a helical torsion bar. Where leaf springs were employed, the longitudinal semi-elliptic configuration was the most favoured. Such springs had a curved leaf or leaves and normally one end is pivot-mounted to the chassis, the other being connected by means of a link-called a 'shackle' - to accommodate the change of length as the spring's curvatures alter with varying loads. The axle is clamped to the spring at or about the middle of its length.
Cantilever Springing
An alternative arrangement favoured by designers of luxury cars in the Edwardian and Vintage era, was to pivot the middle of the springs on the chassis, clamp the axle to one pair of ends and have the shackles at the other ends. This was known as cantilever springing. Other variations tried on the leaf-spring theme were the quarter-elliptic, three-quarter-elliptic and fully elliptic forms. The first of these is (as its name indicates) half a semi-elliptic, carrying the axle at one end and chassis-mounted at the other. The three-quarter-elliptic spring was a combination of quarter and semi, while the fully elliptic was two opposed semis connected at the ends. These last two-hangovers from the horse era had the disadvantage of providing poor lateral location between body and axle, and hence a 'swaying' ride.
Quarter and semi-elliptic are reasonable in respect of lateral location (though far from perfect) but the former are the less easy of the two to endow with adequate flexibility unless the metal is relatively highly stressed. Either the springing had to be firm, as was that of the beam-axle used on the
Frazer Nash sports cars and GN inspiration, or the springs had to be very long. On most leaf-sprung cars the suspension system amounted to little more than a pair of longitudinally disposed semi-elliptic springs, one each side of the chassis, with the axle bridging them. However, one or two manufacturers in search of even greater economies preferred to employ a single transverse semi-elliptic in place of longitudinal springs.
Among these firms was
Ford, using transverse leaf springs from the original
Model T of 1908 until well after the end of World War 2. These springs were attached in the middle to the chassis structure and at the ends, through shackles, to the axle. On the face of it, the single mounted transverse spring had a lot to commend it, in that it was mechanically simple and economical of manufacture and repair. All was not sweetness and light, though, since the central anchorage imparted only limited resistance to swivelling in the horizontal plane. It followed that some additional means of axle location was necessary: it usually took the form of a V-shaped member attached at its apex to the chassis, by a universal joint, and to the axle at its extremities.
A secondary disadvantage was the low resistance to lateral roll of the body on the springs. This was not a serious snag in the days when the cornering abilities of cars were considerably restricted by limited
tyre adhesion and lack of understanding of
handling phenomena. It might never have done for a
Bugatti or an
Alfa Romeo, but the buyers of economy cars such as the Fords probably knew no better and certainly seemed to desire nothing better. For the first decade or two of existence of the
automobile, when performance was low and inter-leaf friction high, the springs were left to function in a fairly uninhibited manner. In those rare instances when performance was high, it was even rarer to find any dampers to modulate their elasticity, though there were pot-type dampers as early as 1902 on the big racing
Mors.
However, it is a physical fact that any mass mounted on a spring (or suspended from it) has a tendency to continue oscillating once it has been disturbed from its position of rest and then released - as happens when a wheel passes over a bump or a pothole. The resulting bouncing tendency of the body became increasingly noticeable as speeds rose and occupants became more comfort-conscious. As an indication of what unrestrained springing could achieve, there is a fascinating story from racing history in the days before World War 1 when Vauxhall used to participate. One of their cars is reputed to have met an undulating bit of road surface at such a speed that the bump spacing coincided with the natural frequency of bounding of the body on its springs. The resulting 'resonance' caused the amplitude of the oscillation to build up to such an extent that the car bounded off the road, over a hedge and into a field.
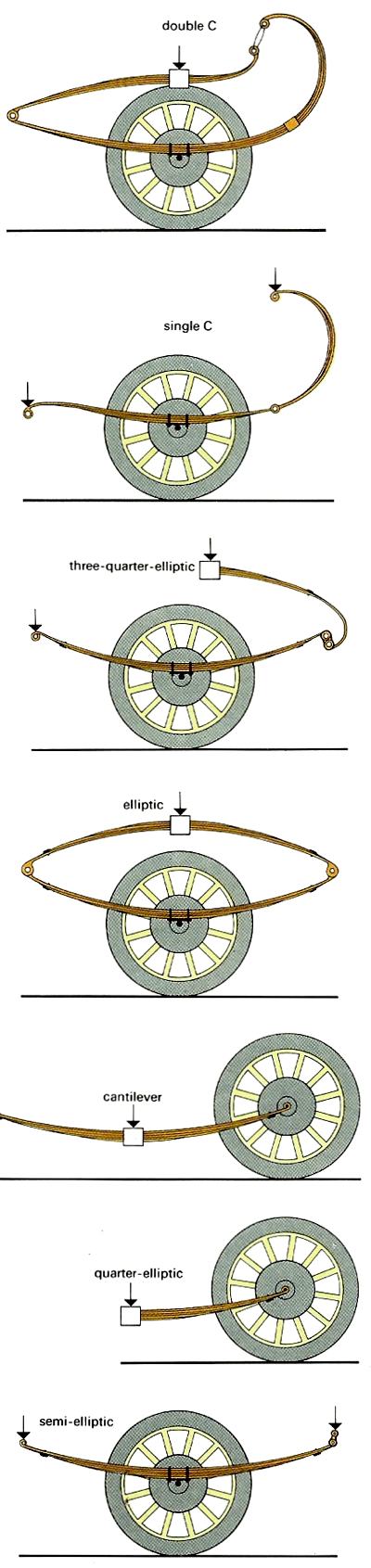
One for the Vintage Car enthusiast - the various types of leaf spring, roughly shown in their correct evolutionary order.
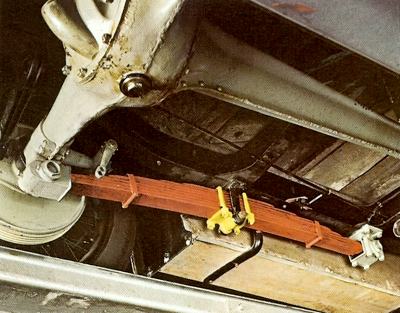
Underbody shot of a 1926 Type 20 Diatto - the advantage of this type of setup over the semi-elliptic was the reduced unsprung weight.
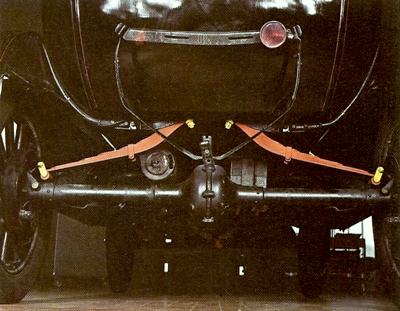
Underneath the 1909 Ford Model T -the transverse leaf spring clearly visible in red.
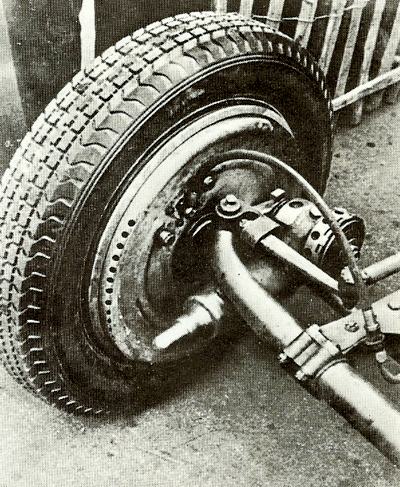
The steered swinging arm angement of the Dubonnet suspension, as seen in a 1935 Alfa Romeo P3. The unsprung weight was low due to the spring and steering linkage being chassis mounted - a huge advantage.

The Panhard Rod was an excellent way of providing lateral location of a beam axle. The rod itself was made as long as possible in order to minimise the arc created by suspension movement.

Mercedes-Benz improved the standard swing axle layout wheny they fitted low-pivoted arms on their 1937 GP car. This layout continued to be improved with the addition of compensating spring to control the fied height of the car and the overall stiffness of the suspension. These days, it is best remembered on the 230SL, 250SL and 280SL.

A Fiat brochure shot showing the swing-axle system as used on the Fiat 128 coupe. The transverse leaf spring fored the major link.
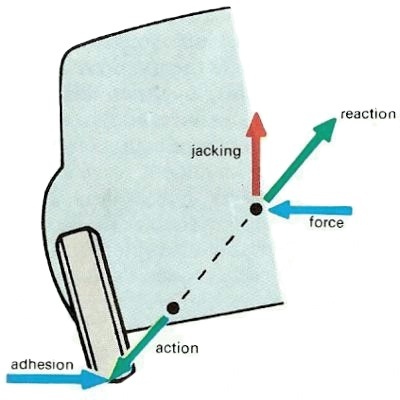
When cornering, centrifugal force is applied through the centre of gravity to the contact patch of the tyre: the reaction results in the car being lifted and, with swing-axle suspension, the wheel tucking under and losing adhesion.

From left to right is positive camber, to negative camber. The correct camber will alllow the maximum amount of radial tyre tread on the road surface during cornering.
|
Friction Dampers
Most car makers had begun, by 1920, to employ some sort of inhibiting devices for the springs. Often they were content to fit the so-called 'snubbers' which served merely to limit the rebound travel of the springs after deflection, and thus prevent any resonance from building up to unmanagable proportions. Next on the scene came the friction damper. In its simplest form this comprised a pair of jointed links, one attached to the chassis and the other to the axle; sandwiched between them at the joint was a disc of friction material, and the connecting bolt could be tightened or slackened to increase or reduce the amount of resistance exerted by the joint. This additional friction in the system damped the natural tendency of the springs to continue oscillating after deflection.
Friction dampers of this type were improved during the succeeding years, to the extent that by the early 1930s some cars had versions that were adjustable by the driver, while seated at the wheel, to achieve the best setting for any particular combination of vehicle loading and road conditions. But even this sophistication was unable to mask the fundamental inadequacy of this method of damping. This was that the static friction between the two components was always greater than the sliding friction. Consequently, the resistance of the system to initial movement was at a maximum when it should have been minimal for the best ride; conversely, bigger impacts received a relatively smaller damping effect. Since car performance was continuing to rise, underlining the friction damper's deficiencies, something better had to be found - something that was more 'velocity-conscious'.
The Hydraulic Damper
The answer lay in the hydraulic damper which, from relatively humble origins in the 1930s (it was, of course, invented much earlier) soon became the general wear that it has remained to this day. Early hydraulic dampers were of the part-rotary type, consisting of a chassis-mounted housing and an external lever arm that was connected to the axle by means of a link. Inside the body was either a part circular space containing a paddle vane operated by the arm, or a cylindrical chamber containing a similarly actuated piston. In each case, movement of the arm caused the fluid in the chamber to be forced through a valve from one side of the vane or
piston to the other. The resistance of the fluid to the forced flow absorbed energy and provided the required damping effect on the spring movement; it was controlled primarily by the size of the valve opening and by the viscosity of the fluid.
However, these dampers had the great operational advantage of being speed-sensitive in that the fluid flowed readily in response to leisurely wheel movements but met with increasing resistance - because of the limited opening size - as the rate of wheel travel rose. Although the piston-type lever-arm damper remained fitted to cars as late as the mid 1970's, it was supplanted in popularity by the telescopic variety. This was just beginning to establish itself immediately before World War 2 and made rapid strides thereafter. In some applications the telescopic damper has advantages, and it can carry a coaxial helical spring (thus simplifying the system), but it cannot form part of the linkage as readily as a lever-arm unit.
Independent Suspension
It was not unknown for the arm of an old-fashioned friction damper to double as a suspension linkage in helping to locate a beam axle, as such as that used by
Frazer Nash. However by the 1930s performance increases and the demand for matching standards of comfort and
handling had begun to highlight the weaknesses of the beam axle and 'cart' springs. Independent suspension had been on the scene for ages before: even the sliding-pillar frontal systems of Morgan and Sizaire-Naudin were not the first, and when Lancia adopted something similar for his 1922 Lambda with a whole host of other independent front suspension (IFS) systems drawn up by his gifted engineer Falchetto.
The interest in independent suspension came about because front
brakes were becoming common, and because
tyres and wheels were getting fat and heavy. It was not just a matter of keeping down the ratio of un-sprung to sprung weight, although independence was a good way of halving it at a stroke: it was a matter of keeping gyroscopic precessions at bay. A hefty
tyre on a large-diameter wheel acts as a flywheel and a gyroscope. If tilted in one plane while spinning it will react by tilting in opposition in a plane perpendicular to that one. Lateral tilt of a beam axle makes both wheels try to precess: if the left wheel rises over a bump, both wheels will steer left. On a rigid rear axle they cannot, but the extra degree of freedom they enjoy on their swivels at the front allows them to fight back. So long as the front springs were stiff, the axle could not tilt much, so the precessional effect was kept within reasonable bounds.
The Wishbone
Any serious attempt to improve the ride by softening the springs, though, led to unacceptable
steering characteristics. This impasse caused car designers to begin a complete rethink on front suspension layouts. Their answer lay in independent suspension, in which the behaviour of one wheel would not markedly affect that of the other. If the geometry of the independent front suspension (or IFS) were designed so that each wheel could travel up or down quite a long distance without tilting much, then the ride comfort could be considerably improved without sufficient processional tendency to affect the
steering adversely. In several of the earlier IFS designs, use was made of a transversely mounted leaf spring which served the secondary purpose of forming part of the wheel-locating linkage; the latter was usually completed by a transverse pivoted arm, usually of the triangulated form (hence the term 'wishbone') to resist the longitudinal forces imposed by braking.
This was a reasonably economical layout in respect of material usage but had the geometrical disadvantage that the length of one of the links was not fixed, since that of a leaf spring of course varies with its curvature which in turn changes with the loadings. The result was some variation in camber angle, which could not only reduce the cornering power of the
tyre but also introduce a precession. A further objection to the transverse spring was its claim to space: IFS encouraged a forward mounting of the engine if cross members could be shifted out of the way, and by using two wishbones the move could be accomplished. This double-wishbone arrangement, with the outboard ends of the wishbones jointed to an upright carrying the hub, is kinematically described as a four-bar linkage, and it has been the most popular form of IFS.
Designers soon learned that the wishbones needed to be neither equal in length nor parallel, and that they needed to consider camber, roll-centre height, steering, ride quality and even spring rates - the characteristics of which could be changed by modifying the geometry of the linkage. This made it popular, though other systems (the Porsche system of twin trailing links and the Dubonnet system of a steered swinging arm, for instance) enjoyed some popularity among designers with objections to
tyre scrub and unsprung weight. The softer springing made practicable by the adoption of IFS brought its own problems, one of which was excessive body roll in cornering.
The Anti-Roll Torsion Bar
This tendency was countered on some cars by the addition of an anti-roll torsion bar-an innovation that was first seen in normal production just before World War 2. An
anti-roll bar consists of a steel rod with angled ends. The straight portion is freely mounted transversely on the body, and the ends are connected to the axle or suspension arms, either directly or through links. If both wheels rise or fall equally, the bar merely rotates in its mountings without twisting. In cornering, though, lateral weight transfer causes the outboard wheel to rise and the inboard one to fall. The ends of the bar therefore move in opposite directions to apply torsion to the bar, the reaction of which resists the roll of the body relative to the wheels.
So effective was the adoption of the
anti-roll bar that it seems strange that it was not earler, and universally adopted by all car makers. But there was a problem, as they reduced the sensitivity of the springing in some circumstances (single-wheel bump, for example) and, unless the front and rear stiffnesses were correctly balanced, the addition could adversely affect the car's
handling characteristics. Moreover, an
anti-roll bar can be caused to oscillate in torsion at its natural frequency, since it is an un-damped spring; if it is a stiff bar, this oscillation gives rise to an unpleasant transverse motion known as 'roll-rock'.
MacPherson Strut Suspension
The double-wishbone layout became very well-established world wide in the first few years following World War 2. Then
Ford put the cat among the pigeons with their
1951 British models, which introduced the MacPherson strut front suspension. This contained no completely new ingredients, its novelty lying in the combination employed. In essence the MacPherson system comprises a telescopic strut (with the stub axle at the bottom), a transverse pivoted arm for location and an
anti-roll bar. The strut is flexibly anchored at its top to the body structure, encloses an hydraulic damper, and usually carries an external coaxial helical spring. The special feature that distinguishes the McPherson from other strut-type suspensions before or after is the use of the
anti-roll bar to triangulate the transverse arms so as to cope with longitudinal loads. This is done by mounting the bar ahead of the arms, inclining its ends obliquely rearward and attaching these to the outboard ends of the arms.
Because of its mechanical simplicity and the reasonable geometry that it provided, the basic strut/arm layout was adopted by many manufacturers. (Although these designs are often referred to as 'MacPherson strut', this designation is inaccurate since they do not incorporate the essential, patented MacPherson integrated
anti-roll bar.) However, there are two potential disadvantages of strut suspension: because of its offset line of action, the strut itself is subjected to bending, which can interfere with its freedom of telescoping, and - in the case of front suspension - the body structure has to be reinforced at the upper front corners to take the loads.
While independent layouts were coming into use at the front, little progress was being made in rear suspension. The combination of 'live' beam-type rear axle, semi-elliptic leaf springs and hydraulic dampers worked well enough in most instances though it had obvious deficiencies. Since it was also economic to manufacture, and provided quite good insulation of the body from <a href="how_it_works_transmission.php" target="_self">transmission</a> and road noise, there was little real incentive to improve it. The main drawbacks of this rather primitive system were only two: leaf springs do not provide very positive location of the axle beam, and the ratio of unsprung to sprung weight is high.
The Panhard Rod
During the 1960s there was a growing tendency - particularly for the higher-performance type of car - to improve the behaviour of the live rear axle by endowing it with some form of positive location. The simplest type of locating device is the Panhard rod. This very old invention is a long transverse bar attached to the axle at one end and the body/chassis at the other; its purpose, clearly, is to limit lateral movement of the axle relative to the body; but because the rod swings in an arc there is still some lateral movement of the axle. Some manufacturers adopted the alternative or additional procedure of restricting slewing of the axle by means of longitudinal links. These served the useful secondary purpose of preventing 'wind-up' of the axle on the springs during heavy braking or acceleration, which forces a flexible leaf spring to adopt a reflex or S shape.
The combination of Panhard rod and longitudinal links certainly locates the axle positively and so can significantly improve
handling characteristics. Any system of trailing links introduce ssome rear-axle steer because their arcuate motions draw each axle end forward in roll or unilateral bump. A better method was devised by Dr Fiedler who went from Horch to BMW in the 1930s and created a torsion-bar rear suspension with transverse links connecting the ends of the axle to the ends of the longitudinal torsion bars, retaining the A frame for lateral location and axle torque reaction at some expense in roll centre height, which had to be above or below the big final-drive housing in the middle of the axle.
The Watt Linkage
The final refinement was introduced by
Bristol in 1958, with a Watt linkage to locate the axle laterally and a simple link to replace the A-frame for torque reaction. The result was complete elimination of rear-axle steer, the axle moving only in a vertical plane perpendicular to the direction of travel of the car, while the roll centre could be at any chosen height. It was a great system for a conventional front-engine/rear-drive car with a live rear axle, but it still suffered from the unfavourable ratio of unsprung to sprung weight. In theory, therefore, it could not provide the optimum riding qualities, although it (and other live-axle layouts) could be made to work surprisingly well for quite modest production costs.
Those who sought independent rear suspension did so with a variety of motives, but accurate
steering and good
handling seemed not to be among them. The swinging half-axle, which Rumpler and Ledwinka propounded in the early 1920s, enjoyed far more popularity than it deserved, mainly due to its adoption by Ferdinand Porsche for everything from the
Volkswagen to the GP Auto Union: it was a particularly convenient and cheap way of integrating the <a href="how_it_works_transmission.php" target="_self">transmission</a> with a rear engine. Front-engined cars with rear-drive and IRS were doomed to the same imperfect system until, in the 1950s, some makers of higher-class cars sought improvement with a modified swing-axle geometry or with a suspension that (as inspired by the 1937 GP Mercedes-Benz) gave independence of tractive torque reaction (resisting which demands stiff springs) and final-drive weight without real independence of the wheels.
The de Dion System
Where rear-wheel drive is employed, either solution enables the heavy final-drive unit to be mounted on the main structure instead of flapping up and down on the springs; the resulting reduction of the unsprung weight is considerable, but the isolation of <a href="how_it_works_transmission.php" target="_self">transmission</a> and road noises and vibration, now given an easier and more direct path to the passenger compartment, becomes much more difficult. For a time there was only one semi-independent layout which achieved lasting success - the de Dion system (invented for de Dion by Trepardoux in the nineteenth century) in which the wheels are connected by a relatively light tubular beam, cranked amidships to clear the fixed final-drive casing. The beam is ideally located (usually by some sort of linkage) so that it can only move bodily up and down and/or tilt laterally; but the ideal is rarely achieved, geometrical solecisms being as common in the location of a dead beam axle as in that of the live variety.
Understanding Camber
Universally jointed half-shafts transmit tractive torque from the differential to the wheels. The de Dion layout has the advantage in cornering on smooth roads or traversing two-wheel bumps that there is no change in the camber angle of the wheels (the angle, if any, between the plane of the wheel and the vertical). This gives more consistent
handling characteristics than when camber variations occur. Over one-wheel bumps, on the other hand, the axle obviously tilts so the camber is effectively changed. Wheel camber has an important influence on
handling since it affects the cornering power of the tyres: a
tyre that is leaning in the direction of a turn exerts more cornering force than one leaning outward. When a wheel leans outward relative to the car it is said to have positive camber, a leaning-in wheel having negative camber.
You might think that, if both wheels have a positive camber, the extra cornering power of the leaning-in inside wheel on a bend would offset the reduced cornering power of the leaning-out outside wheel. This is not true: the inside
tyre is also more lightly loaded owing to the lateral weight transfer caused by centrifugal force, and cannot contribute much to the total cornering power of the pair of tyres. It follows that a pair of negatively cambered wheels generate a higher total cornering force than do their positively cambered equivalents. Even on a de Dion axle this can be arranged with advantage.
Although there were a few exceptions, independent rear suspension in historical terms is mainly a post-war development, and the rapid rise to popularity of front-wheel drive (inspired by the
Issigonis Mini of 1959) has been largely responsible. When there is no final-drive unit to contend with, IRS becomes much simpler and cheaper - though VW and Alfa-Romeo proved that good ride and wheel control could be achieved with a light beam axle. When front-wheel drive came in, swinging rear half-axles went out: there was no need to pivot them on a final-drive casing that was no longer there. Only a few (mostly rear-engined) small cars survived to illustrate the dynamic terrors of a system that looked frightening even when static.
Because of the swing-axle system's inherently poor geometry, satisfactory
handling qualities in cornering could be attained only with difficulty. Unless the system was set up with appreciable negative camber in the static-laden condition (one object of which is to lower the roll centre), the outside wheel tends to 'tuck under' when the car is cornered hard, raising the back of the car (and the roll centre) and causing a marked loss in cornering power. The resulting oversteer can occur quite violently, easily leading to loss of control by the driver. More satisfactory geometry is obtained by mounting each wheel carrier on a 'semi-trailing' arm or wishbone member pivoting about a horizontally skewed axis; the two pivot axes converge towards the rear of the car.
This arrangement requires half-shafts with a universal joint at each end, usually with a splined sliding coupling or other means of accommodating any changes of length that occur with wheel travel. Since the wheel is at an angle to the pivot axis it follows a conical path in moving up and down. Hence there are variations in both the camber angle and the direction the wheel is pointing, leading to some rear-end
steering effects. The layout is therefore a compromise; but it liberates so much space that it is widely used on medium and high-priced cars. Fully trailing arms, with transverse pivot axes, are rarely used for IRS on rear-wheel drive vehicles. One reason for their unpopularity is that the geometry results in greater length variations in the half-shafts, as the suspension operates, than occur with semi-trailing arms.
This phenomenon does not apply to front-drive cars which consequently often feature trailing-arm systems at the rear. Strut-type IRS may have many good characteristics, but it is less easy to install at the rear since the upper ends of the struts encroach undesirably into the body space. The same objection applies to double-wishbone suspension.
Interconnected Suspension
An unconventional suspension layout was known as interconnected suspension, in which there was some sort of link between the front and rear wheels on each side. As a result, the natural frequency in 'pitch' (longitudinal rocking) was made very low, with benefit to the riding comfort, without adverse effect on the suspension except that pitch amplitude may be increased. The two major users of interconnected suspension were
Citroen and long defunct
British Leyland; the former employed it on their small cars and the latter on most of their
front wheel drive models. The Citroen design was the simpler of the two, with the front wheels being mounted on leading (forward-pointing) arms and the rear ones on trailing arms.
Each arm was cranked at its pivot end, to incorporate a downward-projecting lever, and the two levers on each side of the car were connected by rods and an horizontal tension spring unit incorporating hydraulic damping. If a front wheel rose over a bump, it tended to lift that corner of the body. It also applies a force to the spring which in turn reacts on the lever of the rear-wheel arm. This causes the latter to be rotated in the same direction as the front-wheel arm, thus causing the rear end of the body to be lifted also and so keeping it level instead of pitching. If both wheels on one side encountered a bump simultaneously, or if they are deflected upward by body roll in cornering, the resistance of the spring was greater than in the single-wheel bump situation since it was being stretched in both directions. Consequently, the spring could be made very flexible in its response to individual bumps without unduly low roll stiffness.
The Hydrolastic System
British Leyland adopted two more complex systems of this type, both designed by Dr Alex Moulton. The first, the
Hydrolastic system, appeared in 1962 on the
Austin 1100 and was subsequently adopted for a number of other models. In the
Hydrolastic arrangement, each wheel had its own suspension unit comprising a rubber springing element and a fluid chamber with integral damping valves; the interconnection between the front and rear chambers on each side was by means of a fluid-filled pipe. The upward movement of one wheel displaced fluid through one damping valve and along the pipe to the other unit, which is thereby extended to compensate for the compression of the first one. This principle was again refined in the more
Hydragas system introduced in
1973 on the Austin Allegro and later fitted to the 18/22 cars. The rubber side of the suspension units was replaced by gas under compression, to enhance the spring response, and the damping and other details were also improved. The effect was to make possible independent provision for soft ride and good
handling stability.
The Citroen Hydro-Pneumatic Suspension System
For many years the bigger Citroen cars featured an outstanding hydro-pneumatic suspension system in which the individual spring units consisted of chambers containing gas which was compressed (through a diaphragm) by fluid displaced by upward movement of the wheels. The arrangement also incorporated a self-levelling facility to maintain the 'ride height' regardless of the load carried by the car, thus ensuring that full spring travel was available even when the car was fully laden.
The self-levelling was effected by means of an engine-driven hydraulic pump which supplied a valve adjacent to each suspension unit. These valves were actuated by links from the wheel-carrying arms. An increase in the load on a wheel caused upward movement of its arm relative to the body; that movement operated the valve and caused fluid to flow into the unit to restore the original position of the arm. Conversely, a reduction of wheel loading moved the valve the other way and released fluid from the suspension unit. The Citroen and the
Hydragas systems (and the related system on the Mercedes-Benz 450 SEL 6.9) relied on a constant mass of compressed nitrogen to act as a spring.
Another form of pneumatic suspension used a constant volume of gas, and this gave a different character to the ride: constant mass gives a rising spring rate (it gets stiffer as it is deflected) allowing soft springing in normal conditions without excessive wheel travel under heavy loads, whereas the other type gives a virtually constant rate and is no longer employed by any manufacturer.
Also see: How To Guides - Suspension Repair |
Steering and Suspension Repair